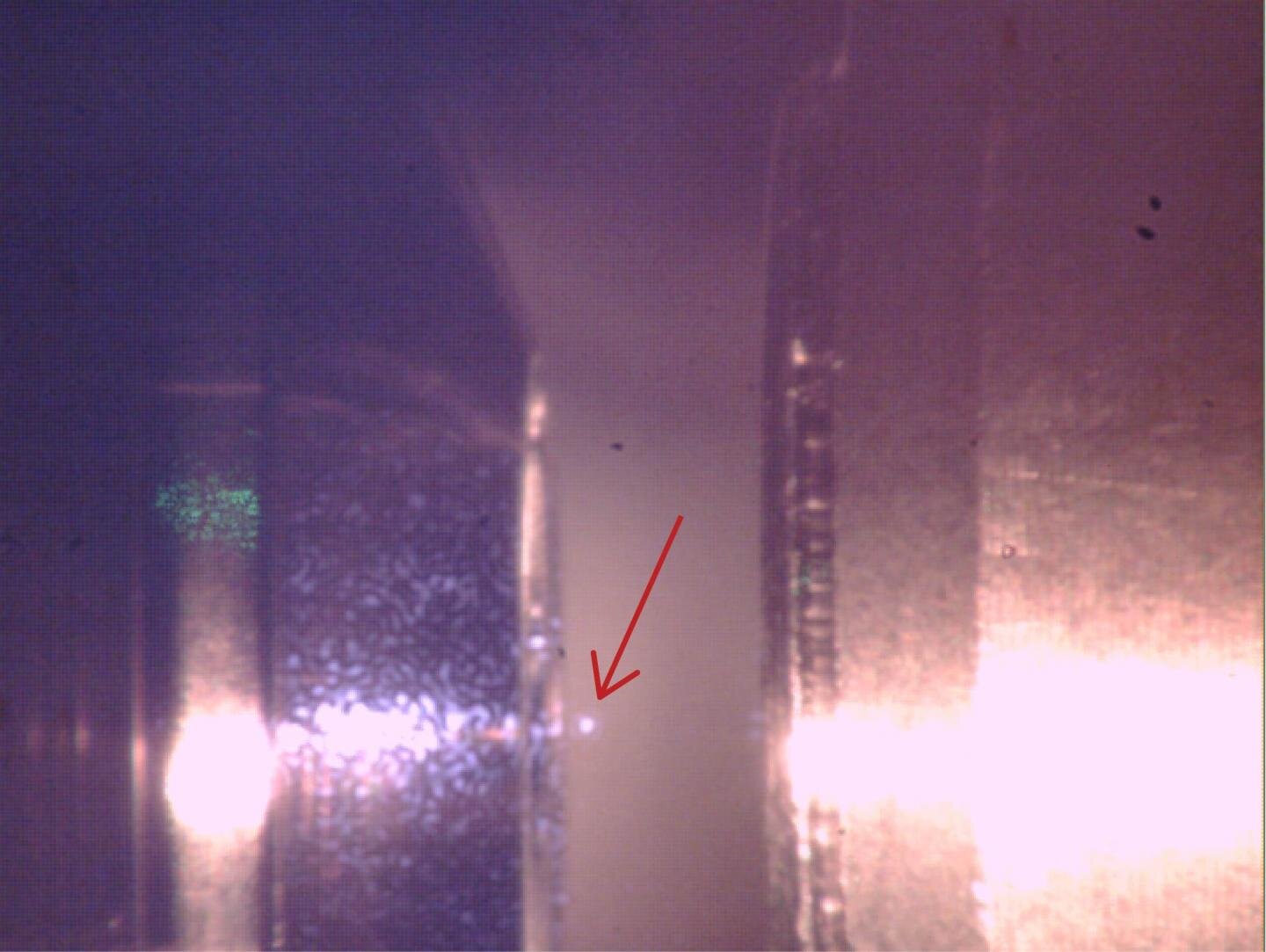
Credit: Lorenzo Magrini/Constanze Bach/Aspelmeyer Group/University of Vienna
There are significant differences between microscopic quantum particles and what we know in ordinary life. Quantum phenomena are usually exceedingly delicate. To examine them, scientists often utilize a tiny number of particles that are properly protected from the environment and kept at the lowest temperatures feasible.
However, thanks to a collaboration between the Austrian Academy of Sciences, the University of Vienna, and TU Wien, it is now possible to monitor and manage a heated glass sphere containing around one billion atoms with unparalleled precision. Its movement was deliberately slowed down until it assumed the ground state of lowest possible energy. His movement was slowed gradually till he adopted the lowest energy level on the ground. The measuring approach came close to exceeding Heisenberg’s uncertainty principle’s limit of precision—physics simply doesn’t allow for any more. The use of unique techniques from control technology to quantum systems has made this possible.
Excellent precision is impossible
This is one of the fundamental principles of quantum theory. The measurement influences the measured object. The Physicist Lorenzo Magrini, the original author of the study at the University of Vienna, explained that Berner Hertz has created a famous thought experiment—the so-called Heisenberg microscope. If you are looking for a very exact measurement of the position of an object in a microscope, you must use light as quickly as possible. But short wavelength means greater energy so that the particle movement is more disturbed.
A particle’s position and movement status cannot be accurately assessed at the same time. The so-called Heisenberg uncertainty principle states that the product of their uncertainties is always restricted by Planck’s constant. However, it can be determined how close one can by nature reach this limit.
This is researched with a glass sphere of fewer than 200 Nanometers in diameter, consisting of around one billion particles by Prof. Markus Aspelmesyer’s team at the University of Vienna, very small, but quite large, as compared with objects normally examined in quantum physics.
With a laser beam, the glass sphere may be maintained. The laser heats the sphere’s atoms, causing the sphere’s internal temperature to increase to several hundred degrees Celsius. This means that the glass spherical atoms are forcefully wobbled around. But in this experiment, the collective motion of the sphere in the laser trap was not analyzed by the wobbling of the individual atoms. According to Markus Aspelmeyer, these two things are entirely different, just as pendular movements on a clock are different from moving individual atoms inside the pendulum.
Quantum control technology
Even though the glass sphere is a macroscopic object, the aim was to exactly regulate the pendulum motion of the glass sphere on a quantum level. This can only be accomplished with a well-designed control system that has been fine-tuned to the experiment. Prof. Andreas Kugi’s team at TU Wien took on this challenge.
“Control engineering is about influencing systems in such a way that they exhibit the desired behavior independent of disturbances and parameter fluctuations,” says Andreas Kugi. “This can be a robot arm, for example, a production line in a factory, or even the temperature of a blast furnace.” The use of current control engineering techniques in quantum systems opens up new possibilities. “However, one also has to face challenges that do not exist in classical system theory and control engineering,” explains Kugi. “In classical control engineering, the measurement has no or negligible influence on the system. In quantum physics, however, this influence cannot be avoided, for very fundamental reasons. We therefore also have to develop novel control engineering methods.”
It’s Success
Using a sophisticated microscopy technique, the light backscattered by the glass sphere was identified as fully as possible. The scattered light analysis determined the sphere’s position in real-time and regularly revised the electrical field such that it permanently counteracted glass sphere movement. Regardless of the fact that it is a very massive object at high temperatures, whose atoms wobble vigorously, it was feasible to slow the whole sphere and bring it into a state of motion that matches the quantum-physical ground state, i.e. the condition of the minimum possible kinetic energy.
Guaranteeing cooperation between physics and control engineering
Lorenzo Magrini added that spatial and kinetic uncertainty must always be taken simultaneously. In total, Planck’s quantum activity was just 1.7 times that of the glass sphere. Planck’s constant would be the ultimate theoretically lower limit since an experiment with an object of this size never before came close to the absolute quantum limit. The experiment’s cinematic energy was just 5 micro-Kelvin, 5 millionths above absolute zero, which was measured. Thus, even when atoms in the sphere are very warm, the movement of the glass sphere in its entirety may be incredibly low temperature.
This achievement demonstrates the enormous potential of this unique combination of quantum physics and control engineering: both research teams intend to continue in this path and use control engineering know-how to perform even better and much more accurately controlled quantum experiments. There are a variety of uses for this, ranging from quantum sensors to quantum information technologies.
Reference:
Research paper: Lorenzo Magrini et al, Real-time optimal quantum control of mechanical motion at room temperature, Nature (2021). DOI: 10.1038/s41586-021-03602-3
- https://www.google.com/amp/s/phys.org/news/2021-07-approaching-heisenberg-limit.amp